By Matthew Gadman, John Florio, Ravi Ravichandran, and Steven Woltornist, King Industries, Inc.
New water-soluble urethane diol resins have been developed to be used as resin modifiers for waterborne coatings systems crosslinked with amino resins. These urethane diols are a multipurpose chemical species that can be utilized to enhance performance properties as well as resolve some of the common issues experienced when formulating waterborne systems.
The above-mentioned resin modifiers are water soluble without the presence of amine neutralizers, surfactants, or co-solvents. Incorporating these urethane diols will thus improve the water solubility of a resin system in a wet paint, subsequently reducing the need for neutralizing amines and additionally allowing for easier resin incorporation with less co-solvent requirements. As resin modifiers, the urethane backbone allows formulators to enhance performance of aminoplast crosslinked coatings by incorporating urethane groups into a crosslinked network without the use of an isocyanate.
This work demonstrates the versatility of these urethane diols and further discusses how they can be used to enhance coating performance by improving corrosion and humidity resistance, as well as increasing hardness while maintaining flexibility.
Introduction
Waterborne Polyester Formulations Crosslinked with Amino Resins
Polyester (PE) resins are often used as a primary binder resin in a range of waterborne (WB) aminoplast crosslinked coating formulations. The flexible nature of the polyester combined with the toughness and good durability associated with amino crosslinks enables these coatings to be used in a variety of direct-to-metal interior and exterior industrial applications. These types of resin systems are often used as baking enamels for general industry as well as for waterborne base coats and primers for can, coil, and automotive applications.
The polyesters commercially available today for waterborne applications are usually saturated polyesters and contain both hydroxyl and carboxyl terminal groups.1 They are typically available as either water-reducible, thinnable, or dilutable resins. Water-reducible resins are supplied in a coalescing solvent, such as 2-butyoxyethanol or sec-butanol, and require the use of amine to fully solubilize the resin in water. In the case of the water-thinnable or water-dilutable polyesters, these resins have already been reduced and supplied partially in a coalescing solvent with amine and water. Thinnable and dilutable resins differ in their water compatibility, where water-thinnable resins are typically more easily thinned via addition of water.
The hydroxyl and carboxyl groups of the saturated polyesters used in waterborne polyester-amino resin formulations serve as the reactive sites available for reaction with aminoplast crosslinkers. Examples of amino crosslinkers typically used include melamine, urea, glycoluril, and benzoguanamine-derived formaldehyde resins.2 Melamine-formaldehyde resins are still a standard selection of crosslinker used in the applications previously mentioned. Although crosslinkers derived from urea monomers are generally faster reacting than those derived from melamine monomers, melamine-formaldehyde resins are often chosen for use due to their superior outdoor durability, chemical resistance, and lower cost.
Formulating with Melamine Crosslinkers
The following terminology is commonly used to differentiate between some of the common melamine-types available in the market: fully, partially, and highly alkylated. These terminologies refer to the method in which the crosslinkers are produced and thus are also indicative of the functionality of the final product.
The functionality of a melamine crosslinker will depend on both the extent of methylolation with formaldehyde and the degree of alkylation with a simple alcohol (e.g., methanol or butanol). Many variations in functionality arise during the synthesis of melamine-formaldhyde resins. However, with regard to methylated melamines, fully, partially, and highly alkylated resins are generally characterized by the amount of methylol, methoxymethyl, and imino (NH) functional groups (Figure 1).
When formulating a coating with a hydroxyl-functional polymer using a melamine crosslinker, it is ideal to have equal amounts of available hydroxyl groups and methoxymethyl groups to optimize crosslinking. With respect to fully methylated, or hexa(methoxymethyl)melamine (HMMM), type resins, the equivalent weight of the crosslinker should theoretically be about 80 g/eq. However, this number is somewhat misleading and, in some cases, not necessarily accurate. Two important characteristics of amino resins to note include molecular weight distribution and the resin’s tendency to self-condense in aqueous environments.
First, regarding the molecular weight distribution, an HMMM crosslinker is likely to contain only 60–65% monomer with the rest being mostly dimers and trimers along with small amounts of other higher oligomer types. This can become troublesome when formulating based on theoretical equivalent weights, especially if the molecular weight distribution is unknown.
Formulating aqueous amino resin formulations then becomes more challenging when considering that the crosslinking reactions between hydroxyl and methoxymethyl groups are always competing with the self-condensation reaction of the melamine crosslinker. The self-condensation reaction begins with the hydrolysis of the methyoxymethyl groups, followed by demethylolation and the subsequent release of formaldehyde (Figure 2).
Due to these variables, it can be beneficial to begin formulating by evaluating a resin’s crosslinking capability with a melamine through experimentation rather than using a theoretical equivalent weight to ensure that hydroxyl-methoxymethyl crosslinking is optimized.
Necessity and Effects of Amine in PE/HMMM Coatings
In terms of stability, fully alkylated melamine-formaldehyde resins are inhibited by amines, where reactivity is linearly proportional to the pH of the system. In the case of high imino-type crosslinkers, the presence of amines will have less effect on cure response.3 The differences in stability enable formulators to use HMMM crosslinkers for one-component (1K) systems.
The need for the presence of amines in WB PE/HMMM formulations is thus two-fold, requiring to first reduce and solubilize the saturated polyester in water and then to stabilize the HMMM crosslinker to prevent the melamine from undergoing self-condensation via hydrolysis. However, amines can be problematic for reasons other than being difficult to work with due to their high volatility.
A plethora of issues arise when residual amine remains in the film after cure. Some amines tend to react with the formaldehyde byproduct of melamine self-condensation, forming color bodies and thus causing discoloration issues such as yellowing. Residual amine will also increase the coatings polarity, making it more susceptible to moisture attack and, therefore, decreasing the coatings weathering durability, especially in the case of humidity resistance. Furthermore, it would be logical to assume that a film that is more susceptible to moisture attack would also allow water to easily penetrate the film’s surface, weaken the integrity of the coating, and attack the substrate. This notion would thus indicate that residual amine could reduce the corrosion resistance of a film coated over steel or other metal substrates.
Polyurethane Coatings
Polyurethane coatings produced through polyisocyanate crosslinking will usually provide the robust performance with superior exterior durability properties that a formulator seeks. For instance, in comparison to melamine crosslinks, urethane crosslinks are generally more hydrolytically stable and more resistant to hydrolysis under acidic conditions, allowing for superior environmental etch resistance.4
However, there are still disadvantages when using polyisocyanates. Not only are polyisocyanates generally of a high cost, but they can also pose safety hazards. Prolonged exposure to these materials can be very detrimental to a worker’s health, causing serious skin damage with the potential for subsequent sensitization.5
Polyisocyanates are additionally quite difficult to use, particularly with regard to formulating aqueous systems. Polyisocyanates will react with water producing amine and CO2 byproducts. The amine will react with another isocyanate group rather quickly, forming a urea linkage. Not only is this an issue with formulating stable systems, but it is also detrimental during the curing process as gassing or foaming can often be observable in the cured material, and it can also reduce film gloss.
Urethane Linkages in Melamine Coatings
An ideal situation would be to synergize these technologies and reap the benefits of the lower cost and better stability associated with melamines and the superior durability associated with urethane linkages. There have been a variety of approaches proposed to incorporate carbamate groups into the backbone of melamine coatings in an attempt to obtain a hybrid melamine-urethane crosslink coating. Nonetheless, these approaches have exhibited copious challenges inclusive of cost effectiveness and poor reactivity.
For instance, by using triazine as the foundation of the resin, tris(alkoxycarbonylamino)triazine crosslinkers, or TACT, were developed as a formaldhyde-free melamine crosslinker resin.6 When catalyzed properly, this crosslinker is said to be capable of crosslinking with trifunctional epoxy resin to provide a urethane-linked backbone. Not only does this technology struggle due to high cost but also presents issues with reactivity. Other methods of incorporating urethane groups in the backbone of a melamine crosslinked network are by crosslinking a polyurethane dispersion with a melamine. Nonetheless, this technology also has issues pertaining to reactivity as the reactive sites on many of the commercially available polyurethane dispersions are often tertiary carboxyl groups that tend to have a relatively sluggish reaction with melamine crosslinkers.7
Another method of integrating urethane linkages into the backbone of a melamine crosslinked network is through the use of a urethane diol resin. The hydroxyl functionality of these diols will allow for reaction with the melamine via a transetherification reaction between the hydroxyl and alkoxylmethyl groups of the urethane diol and melamine, respectively (Figure 3).
The urethane diol resins described will allow for incorporation of urethane groups in the backbone polymer matrix of melamine crosslinked coatings without the use of polyisocyanates or polyurethane dispersions. Moreover, these urethane diol resins are not produced using isocyanate chemistry and thus will neither contain residual isocyanate nor pose a risk of urethane deblocking or degrading to produce free isocyanates.
Pursuit of Non-VOC Coatings: The Rise of Aqueous Formulations and Their Limitations
An increase in the use of aqueous formulations began in the 1990s as an attempt to address government regulations being placed on volatile organic compound (VOC) emissions. The automotive industry, for example, has remained steadfast in its efforts to reduce the immense quantity of VOCs emitted during application of automotive coatings by using waterborne automotive primers and basecoats. This has subsequently pressed coatings formulators and manufacturers to develop more aqueous coatings formulations.
An optimal scenario would be to formulate a homogenous, stable, aqueous formulation that provides good flow and leveling with very low levels or—more favorably—completely free of VOCs. As previously mentioned, many aqueous formulations will contain a coalescing solvent to aid in solubilizing resins in water to aid in compatibility as well as to provide good film formation. For example, ethylene glycol monobutyl ether (EGBE) is a rather popular
co-solvent used to reduce and compatibilize saturated polyesters in water.
While VOC levels are largely decreased when using a water-based system, toxicity of selected coalescing solvents should not be disregarded. A somewhat recent petition in 2015 was submitted to the Environmental Protection Agency (EPA) to remove EGBE from the EPA’s Emergency Planning and Community Right-to-Know Act (EPCRA). The EPA denied the petition for removal from EPCRA after concluding that it can be rationally predicted to cause serious or potentially even irreversible chronic health issues with major concerns for liver toxicity and hematological effects.8
It should not be overlooked that formulating aqueous coatings can be quite challenging, especially when compared to solvent-based coatings. When formulating a solvent-based system, a formulator will have the option to select a solvent with physical properties that are best suited for the formulation requirements. Selecting the proper solvent will enable a formulator to have better control over formulation characteristics such as cure time, flow and leveling, and viscosity. Although solvent is tolerated and is often necessary to formulate aqueous coatings systems, water-based systems still significantly limit the previously mentioned options offered by a variety of solvents.
Urethane Diols for Modifying Aqueous Formulations Crosslinked with Amino Resins
The urethane diols of topic are completely water soluble without the use of amine neutralizers or co-solvents. Incorporating these diols into aqueous amino resin crosslinked coatings will thus inherently reduce amines and coalescing solvents that are normally required to prepare a homogenous, stable aqueous system with good flow and leveling.
Urethane diols have a range of physical properties inclusive of but not limited to variations in hydroxyl value, solid content, and viscosity. Physical properties of the diols referenced in the subsequent experimental section are presented in Table 1.
Experimental
The following experiments (I–IV) serve as examples demonstrating the many benefits that can be attained when using these diols as modifier resins.
Experiments I and II investigate crosslinking capabilities of urethane diols with an HMMM crosslinker and reactivity differences of primary vs secondary hydroxyl functional diols, respectively. The potential of using urethane diols as a method of improving acid etch resistance of aqueous amino crosslinked coatings is explored in Experiment III. Film property evaluations using a newly developed urethane diol as a modifying resin in aqueous polyester-HMMM formulations are included in Experiment IV.
Physical properties of the saturated polyesters used in Experiments III and IV are detailed in Table 2.
All formulations involved in these experiments were mixed via high-speed dispersion using a high-speed mixer from FlackTek Inc. Films were prepared over polished, bare cold rolled steel (CRS) and applied via draw down. All films tested were given a ~10 min flash at room temperature (RT) prior to bake.
Experiment I: Crosslinking Capabilities of Urethane Diols with Amino Resins
Diol B was reacted with a fully methylated melamine-formaldehyde (HMMM) with varying ratios of urethane diol to HMMM. Systems were evaluated for cure response at relatively low temperatures to determine the solids required to achieve optimal crosslinking. Crosslinking of cured films was assessed by MEK resistance.9 Dry film thicknesses (DFT) of the films were maintained between 1.6–1.8 mil.
All systems consisted of only the urethane diol, HMMM crosslinker, a flow and leveling agent, acid catalysts, and equal amounts of water (40% on total resin solids). The Diol B-HMMM systems showed good cure response at relatively low temperatures with moderate catalyst levels. Decreasing the cure temperature to below 100°C required high catalyst loading. Results are shown in Figure 4.
Optimal crosslinking of Diol B with HMMM was achieved with a 50/50 mix ratio based on solids. This data suggests that the melamine can be assigned a hypothetical equivalent weight of nearly 160–170 g/eq. when reacting with a urethane diol.
Experiment II: Reactivity of 1° vs 2° Hydroxyl Functional Urethane Diol
Reactivity of these urethane diols was evaluated to determine if resins containing less sterically hindered hydroxyl groups will more readily react with an HMMM crosslinker. Diol B has a mixture of primary (1°) and secondary (2°) OH groups but the latter predominates rather significantly. Only 1° OH functional groups are present in Diol C.
The urethane diols and the HMMM crosslinker were the only resins present in the aqueous systems. Each of the two diols was solubilized in water and precatalyzed with an amine blocked acid catalyst. A flow and leveling agent was then incorporated followed by the HMMM crosslinker. Water content was maintained at 40% on total resin solids.
Cure Response
The ratios of HMMM/OH equivalence were held constant between the two systems using the hypothetical HMMM eq. wt. experimentally determined in Experiment I. The mix ratios of the Diol B/HMMM and Diol C/HMMM systems were 50/50 and 47/53 based on resin solids, respectively.
Cure response was evaluated by testing the MEK resistance of the baked films once cooled to RT. The Diol C system was significantly more reactive with HMMM than Diol B. Results are shown in Figure 5.
Thermal Gravimetric Analysis
The following resin systems were analyzed for weight loss via thermal gravimetric analysis (TGA): 1. HMMM Only, 2. Diol B/HMMM, and 3. Diol C/HMMM. The systems were evaluated with urethane diol to HMMM mix ratios of 0/100, 50/50, and 47/53, respectively. The three systems were catalyzed with 1.2% p-TSA on total resin solids using an amine blocked acid catalyst.
EGBE was needed to compatibilize the system that contained only the HMMM resin. Equal amounts of coalescing solvent were added to all three systems. All formulations contained just over 62% total solids.
The weight loss of the HMMM crosslinker is likely due to the evolution of water, methanol, formaldehyde, and nitrogen-based compounds. The Diol C system repeatedly exhibited the least amount of weight loss and contained more parts melamine than the Diol B system. A possible explanation for this is that the Diol C/HMMM reaction is occurring at a lower temperature than the melamine self-condensation reaction.
Systems were evaluated using a 10°C/min heat ramp to 150°C followed by a 90-min isotherm. Data was collected by using a Q50 TGA from TA Instruments. The TGA curve is shown in Figure 6.
Experiment III: Acid Etch Resistance of Diol D Modified White Pigmented Polyester Coating
$1
Acid etch resistance of a urethane diol modified white polyester formulation crosslinked with HMMM was evaluated using Diol D as a modifier resin. The component breakdown of the grind is shown in Table 3, and the letdown formulation is shown in Table 4a. Formulation characteristics are presented in Table 4b.
Additional melamine was added to the Diol D modified formulation to account for the increase in hydroxyl groups after incorporating the lower molecular weight diol. The ratio of Diol D to HMMM was determined by using the experimental eq. wt. determined in Experiment I. Pigment to binder ratio was held constant for the control and Diol D formulations.
Films were baked at 130°C for 30 min. Both the control and Diol D modified systems were adequately cured as determined by MEK resistance. Each of the films achieved 100+ MEK double rubs.
Acid resistance was tested by subjecting the films to a diluted solution of sulfuric acid (H2SO4) at elevated temperatures. Equal amounts of a 20% H2SO4 solution were placed onto the film in three spots of equal surface area. Test areas were covered, placed in an oven at 60°C, and evaluated in 15-min intervals. The acid solution was rinsed off the panel using dIH2O after the films were near RT. Excess acid or dIH2O was removed by lightly pressing with a towel. Results are presented in Table 5.
Experiment IV: Diol A Modifications of WB PE Crosslinked with HMMM
Formulations: Clear Water-Reducible, Clear Water-Dilutable, and White Water-Thinnable PE
Diol A was evaluated as a modifier resin in three polyester systems. Formulations included a clear water-reducible PE (Formulation I), a clear water-dilutable PE (Formulation II), and a white pigmented water-thinnable PE (Formulation III).
All formulations were crosslinked with HMMM. Modified formulations were formulated to have 8% urethane diol modification based on solids on total resin solids. The melamine content in the modified systems was increased to account for the rise in available hydroxyl groups incorporated upon addition of the lower equivalent weight urethane diol modifier. The ratio of Diol A to HMMM is based on the HMMM eq. wt. experimentally determined in Experiment I. The ratio of HMMM per part polyester was held constant between the control and the modified systems in all three formulations. The pigment/binder ratio was held constant between both systems in Formulation III.
The component breakdown and formulation characteristics of Formulations I, II, and III, are given in Table 6a-b, Table 7a-b, and Table 8a-b, respectively. The grind formulation used for Formulation III is presented in Table 3.
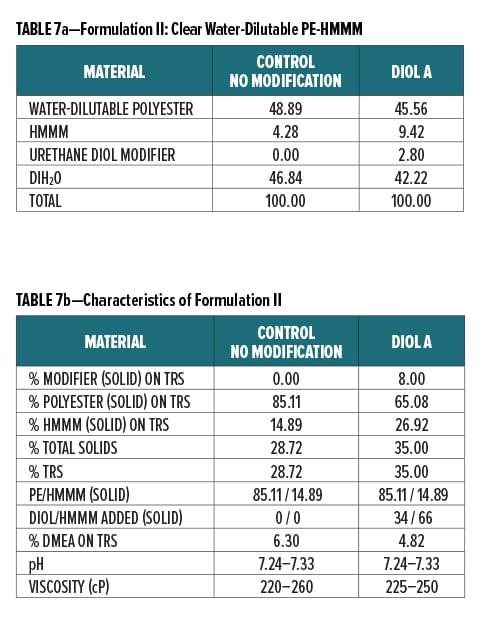
Amine Required for Neutralization
The effects on pH were observed for modifying Formulations I, II, and III with Diol A.
A titration curve for Formulation I is shown in Figure 7. The Diol A modified formulation required less DMEA for neutralization and exhibited overall better water compatibility with less haze present at lower amine levels. Images of the control and the Diol A systems at the start of the titration are shown in Table 9.
Additional amine was not required to neutralize Formulations II and III. Systems modified with Diol A contained a lesser amount of the polyesters and thus subsequently contained less DMEA. Extra DMEA was added only to the Formulation III systems. The amounts of amine required for neutralizing Formulations II and III to the desired pH ranges are given in Table 10.
Solids, Water, and VOC Content
The control and Diol A modified formulations were evaluated at equal viscosities in Formulations I, II, and III. The viscosities of the unmodified and modified systems were reduced using deionized water (dIH2O). The only VOCs present in the formulation are from the polyesters used in the formulation.
Viscosity differences between systems were no greater than 40 cPs. Viscosities were determined using an AR1000 rheometer from TA Instruments (2° steel cone, T=25°C, Shear Rate=100 s-1). Solids comparisons of the control and Diol A modifications are in Figure 8. A comparison of solvent content is presented in Figure 9.
Film Properties
Film properties were evaluated for all three of the aqueous polyester-HMMM formulations. Test specifications for evaluating Formulations I, II, and III are given in Table 11. Film properties tested include MEK resistance, gloss measurements,10 cross-hatch adhesion,11 pendulum hardness,12 pencil hardness,13 impact resistance,14 GE impact-flexibility,15 and mandrel bend.16
Formulations with Diol A provided comparable to improved gloss and MEK resistance in Formulation I. Formulations II and III were relatively similar in terms of these properties. All films showed 100% adhesion. Results are given in Figure 10.
In all three formulations, Diol A modifications provided a significant increase in film hardness without any observable loss of flexibility. Both the control and Diol A systems exhibited excellent flexibility properties. All flexibility tests were evaluated to their maximum testing capabilities for both systems in all three of the formulations. Results are shown in Figures 11 and 12.
Moisture Resistance
The clear polyester–HMMM formulations were evaluated for humidity resistance17 upon exposure to Cleveland humidity conditions. The humidity chamber was maintained at 70°C/100% relative humidity. Systems modified with Diol A provided an overall superior resistance to moisture attack in both Formulations I and II.
The control and Diol A modification both developed a severe haze in Formulation I. The Diol A systems showed improvements in recovery of gloss, hardness, and appearance following moisture attack. Results are presented in Table 12 and Figures 13–14.
In Formulation II, a white-yellow haze appeared around the edges of the control but not in the film of the Diol A system. Both systems showed good gloss after exposure. The modified formulation additionally exhibited improvements in recovery of hardness and color. Results are shown in Table 13 and Figures 15–16.
Salt Fog Resistance
The clear polyester-HMMM formulations were evaluated for corrosion resistance18 following exposure to salt fog conditions.19 Films were scribed vertically with a length of approximately 7 cm. In both Formulations I and II, systems modified with Diol A displayed an overall superior resistance to salt fog.
In Formulation I, the control developed blisters around the edges of the scribe during early stages of testing; corrosion quickly followed. Results are presented in Table 14.
In Formulation II, the Diol A modified systems provided improved resistance to salt fog conditions. The control formed a white haze around the edges of the scribe and had more of a tendency to corrode. The results for Formulation II are shown in Table 15.
Age Stability
The stability of Formulation I was evaluated after being aged at RT, 50°C, and 60°C. The effects on pH, viscosity, pot appearance, film gloss, and cure response were explored for both the control and Diol A modified polyester-
HMMM formulations.
The Diol A modified system exhibited overall better heat aged stability compared to the control.
Sample bottles were sealed to prevent volatiles such as neutralizing amine from escaping. All heat aged pots were cooled to RT prior to testing.
The control was found to be significantly hazier when removed from the 50°C and 60°C storage ovens. Results are presented in Table 16.
The pH and the viscosity of the control and Diol A modified formulations both decreased upon aging. The pH of two systems appeared to decrease at a similar rate during the three days of testing. However, the viscosity of the control system exhibited a more significant change in viscosity than the Diol A modified formulation. The larger decrease in viscosity could potentially be due to poorer water compatibility of the unmodified resin system when at a more neutral pH. This could cause the resin system to be less likely to exist within the water phase of the aqueous system and subsequently result in a less homogenized solution. Results are shown in Figures 17–18.
Gloss and cure response of films prepared from paints that had been aged for three days were compared to those that were prepared when the paint samples were initially made. The control formulation exhibited a much more significant change in gloss vs the Diol A modified system. The heat aged control samples additionally displayed a more severe decrease in MEK resistance compared to the Diol A modified formulation. Results are shown in Figures 19–20.
Conclusion
This work demonstrates that newly developed urethane diols can be used to incorporate urethane groups into the polymer matrix of an aqueous melamine crosslinked coating. This was achieved without the use of an isocyanate or polyurethane dispersion.
Crosslinking capabilities of urethane diols with amino resins were explored. Urethane diols containing primary hydroxyl functionality were confirmed to be more reactive with an amino crosslinker than diols with secondary hydroxyl groups. Furthermore, this work shows the potential for using these urethane diols as modifiers to improve acid resistance of melamine baked films.
The full benefits of using urethane diols were demonstrated by showing the effectiveness of Diol A as a resin modifier in aqueous polyester formulations crosslinked with a melamine resin. Such benefits of using Diol A included increased formulation solids; decreased co-solvent and amine content; improved hardness while maintaining flexibility, enhanced moisture, and corrosion resistance; and better heat-aged stability.
Acknowledgments
The authors would like to thank Michael Emmet for his expertise and continuous dedication to this work. Additionally, the authors would like to thank King Industries, Inc. for permission to publish.
References
1. Jones, F.N., Nichols, M.E., and Pappas, S.P., Organic Coatings: Science and Technology, 4th ed. John Wiley & Sons, Inc.: Hoboken, NJ, 148-149, 2017.
2. Florio, J.J. and Miller, D.J., Handbook of Coatings Additives, M. Dekker: New York, 32, 2004.
3. Blank, W.J., “Reaction Mechanism of Melamine Resins,” presented at Waterborne and High Solids Coatings Symposium, New Orleans, 1979.
4. Wicks, Z.W., Jones, F.N., and Pappas, S.P., Organic Coatings: Science and Technology, 3rd ed., John Wiley & Sons: Brisbane, 601, 1994.
5. Thomas, H. and Hess, R. (Eds.); Waechter, F., (Translator), Toxicology of Industrial Compounds, Taylor & Francis: London, 151, 1995.
6. Essenfied, A. and Wu, K.-J., “A New Formaldehyde-Free Etch Resistant Melamine Crosslinker,” presented at Waterborne, High-Solids, and Powder Coatings Symposium, Feb. 5-7, 1997.
7. Tramontano, V.J. and Blank, W.J., “Crosslinking of Waterborne Polyurethane Dispersions,” J. Coat. Technol., 67, 848, 89-99 (Sept. 1995).
8. 80 FR 60818, Proposed Rules, ENVIRONMENTAL PROTECTION AGENCY (EPA), 40 CFR Part 372, Ethylene Glycol Monobutyl Ether; Community Right-To-Know Toxic Chemical Release Reporting.
9. ASTM D4752–modified, Rub with MEK Soaked Cloth Attached to 2 Pound Ball Peen Hammer.
10. ASTM D2457–Standard Test Method for Specular Gloss of Plastic Films and Solid Plastics.
11. ASTM D3359–Standard Test Methods for Measuring Adhesion by Tape Test.
12. ASTM D4366–Standard Test Methods for Hardness of Organic Coatings by Pendulum Damping Tests.
13. ASTM D3363–Standard Test Method for Film Hardness by Pencil Test.
14. ASTM D2794–Standard Test Method for Resistance of Organic Coatings to the Effects of Rapid Deformation (Impact).
15. FED-STD-141D- FEDERAL TEST METHOD STANDARD: PAINT, VARNISH, LACQUER AND RELATED MATERIALS: METHODS OF INSPECTION, SAMPLING AND TESTING; Method 6226.
16. ASTM D522–Standard Test Methods for Mandrel Bend Test of Attached Organic Coatings.
17. ASTM D2247–Standard Practice for Testing Water Resistance of Coatings in 100% Relative Humidity.
18. ASTM D1654–Standard Test Method for Evaluation of Painted or Coated Specimens Subjected to Corrosive Environments.
19. ASTM B117–Standard Practice for Operating Salt Spray (Fog) Apparatus.
Presented at the American Coatings Conference, April 9–11, 2018, in Indianapolis, IN.
CoatingsTech | Vol. 16, No. 2 | February 2019